TL;DR
In this week’s subscriber issue we took a rapid tour of the quantum computing industry and highlighted startups and big-tech players taking different bets in the space. In today’s issue, we’ll dive a little deeper into the science of quantum computing and discuss superconducting quantum computers. Superconducting logic devices, based on Josephson junctions, have been investigated for decades as a post-CMOS technology for classical computing but have only found their niche with the advent of quantum computing. It is not yet clear whether superconducting circuits will become a lasting technology for quantum computing, but superconducting logic will undoubtedly find a variety of new applications.
A Tour of Quantum Computing
Quantum computing holds out the promise of computational capabilities not feasible with classical computing. Famously, these include Shor’s algorithm for polynomial time factoring and more efficient simulations of quantum systems. Quantum computers represent states with qubits, two level quantum systems. Qubits, unlike standard bits, can exist in a superposition of their two states (which are traditionally represented by |0〉and |1〉). Qubits can be entangled, allowing the states of two different qubits to have a higher correlation than would be possible classically. Entanglement provides a new computational resource, which can be leveraged to speed up algorithms in ways that were not possible before.
Quantum computations are represented as quantum circuits which apply a series of transformations to qubits. The diagram below shows a sample quantum computation. The depth of a circuit is the longest path in the circuit and is a measure of the complexity of a quantum calculation (source).
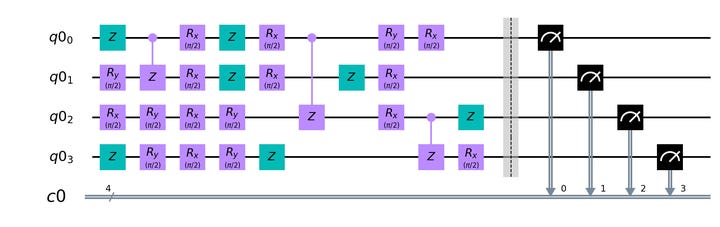
Unlike classical computing, where silicon has maintained dominance over technologies for the last several decades, quantum computing is a nascent industry with considerable uncertainty over the right technologies for scalable quantum computers. There are currently multiple competing substrates for quantum computers. The furthest along is likely superconducting electronic circuits, which implement qubits using superconducting circuits, but other core technologies include ion traps and photonic quantum computing, which implement qubits with trapped ions and photons respectively. We dive deeper into superconducting quantum computers in today’s post since superconducting qubits are currently the leading technology for quantum computers (although there are many competing technologies in the race!).
Superconducting Qubits
Superconducting quantum computers were famously used by Google to achieve the milestone of quantum supremacy, performing a calculation which no classical computer could (source). Superconducting qubits use the technology of Josephson Junctions which connect two superconductors through a weak link (often an insulator, but can also be a non-superconducting metal, or physical constriction). Current passes through the weak link by quantum tunneling, a quantum phenomenon where electrons can pass through small physical barriers.
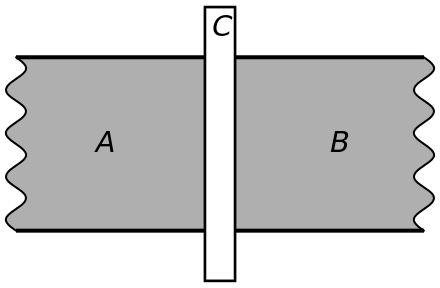
As circuit elements, Josephson junctions have properties distinct from those of more classical circuit elements. The first diagram below shows the circuit diagram representation of a Josephson junction. Josephson junctions have long been explored as a potential substrate for novel computing devices. Superconducting logic constructs logic gates from Josephson junctions. These superconducting logic systems could potentially have very low power consumption and high operating speeds up to 770 GHz! (Source.) Early work considered superconducting logic systems as a potent successor to CMOS systems, but the continuing improvements from Moore’s law have kept superconducting logic from really finding their place in mainstream classical computing. Given the challenges facing Moore’s law, superconducting logic may well have future applications even for classical computers.
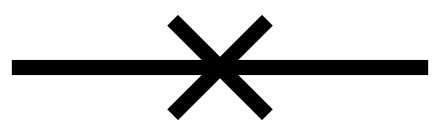
Quantum computing has proven to be the first major application of superconducting logic, with Josephson junctions crucial building blocks for superconducting qubits. The charge qubit represented below represents different states of a qubit according to the number of Cooper pairs (a pair of electrons bound together at low temperatures) that have tunneled onto the superconducting island (the superconducting region shared between the Josephson junction and the capacitor and delineated with a square below). Different qubit states correspond to different levels of charge on the superconductor; charges are quantized at the quantum level, which maps neatly onto the discrete levels needed for the states of a qubit.
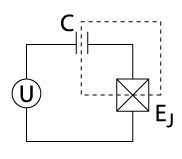
The number of Josephson junctions roughly corresponds to the computing power of the quantum device. Manufacturing techniques for superconducting logic have steadily improved and the maximum number of Josephson junctions on a single device has increased exponentially as the diagram below shows. That said, superconducting quantum computers have not become exponentially more powerful as a result since error rates for these devices are still high.
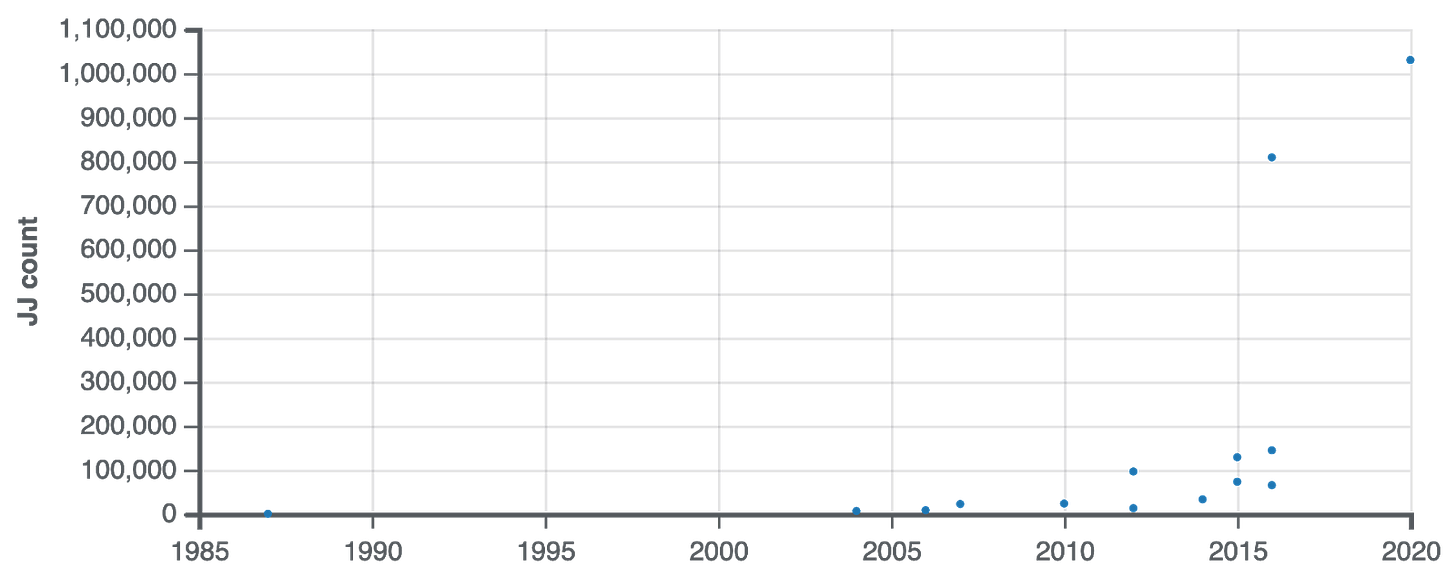
Physical Qubits, Logical Qubits, Error Correction, and Quantum Volume
The difference between physical and logical qubits is fundamental for scalable quantum computing. The charge qubit we saw earlier is an example of a physical qubit implemented with a superconducting circuit.
To understand the need for logical qubits, we first note that quantum computing calculations rapidly accumulate error. As a result, it is not currently possible to run large quantum calculations for many applications. Theoretically, we know there exist quantum error-correction protocols which could allow noise to be managed so that arbitrarily large computations can be run. These protocols require the implementation of logical qubits which would combine multiple physical qubits into one logical unit with better error handling. Today’s corporate players have succeeded in building systems with 50-100 physical qubits, but have not successfully built large systems of logical qubits. Researchers have dubbed the quantum computers that exist today noisy intermediate scale quantum (NISQ) systems and believe that NISQ devices can still be used to implement meaningful algorithms (source).
Comparing different quantum computers to one another is notoriously difficult. The new metric of quantum volume has been proposed as a measure of the capability of a quantum computer and measures a combination of the number of qubits available and the number of calculations possible.
Cryo-CMOS Circuits
One of the largest challenges for superconducting quantum computers is designing control circuits that operate at the low temperatures needed for superconducting logic. Classical circuits typically dissipate heat, disturbing the superconducting logic. Recent advances in circuit design by Google engineers have designed CMOS circuits that bypass some of these heat dissipation issues (source).
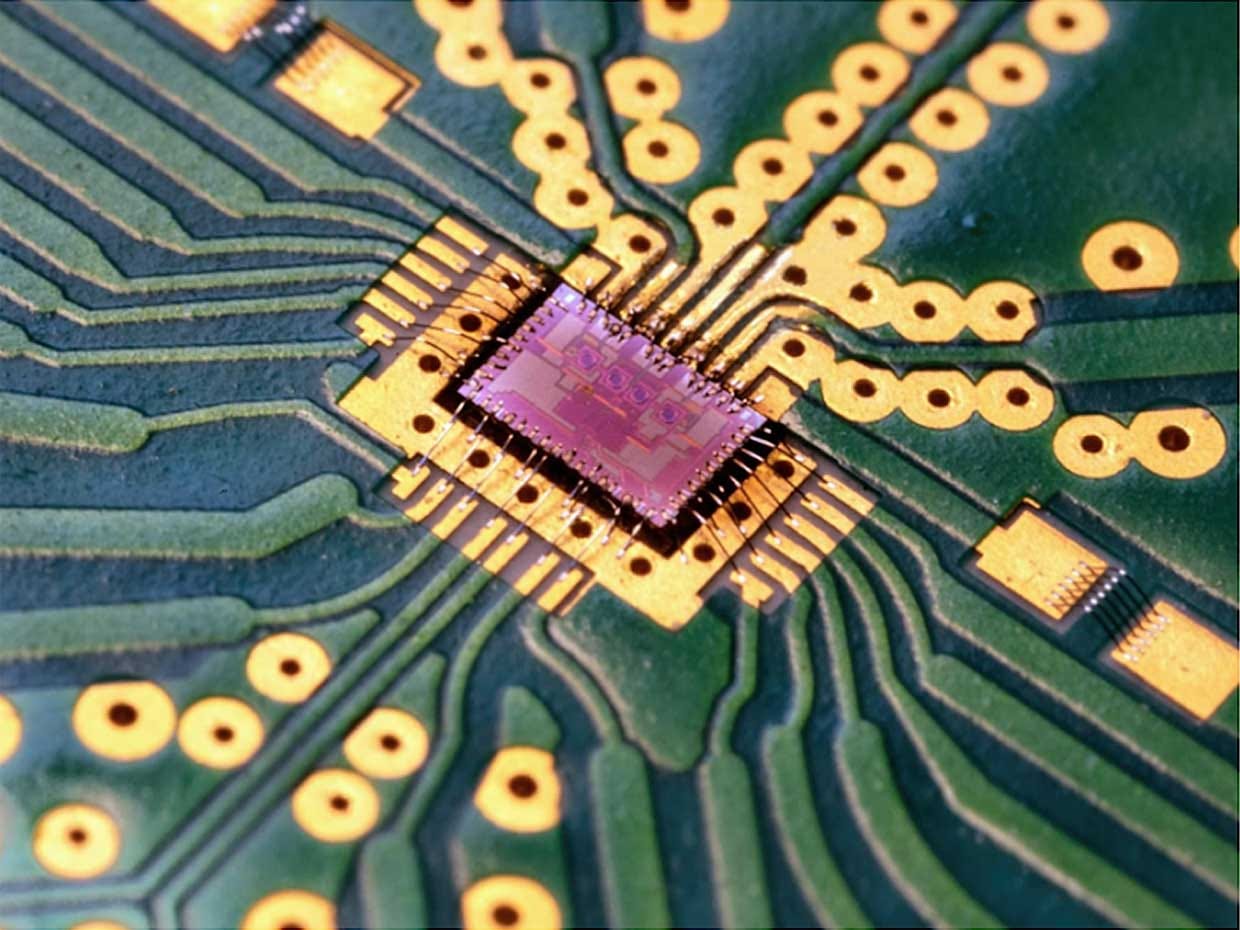
Discussion
Josephson junctions and superconducting logic play a commanding role in today’s leading quantum computers. These systems bring exotic capabilities such as very high switching rates and very low power consumption to the table. Engineering techniques for manufacturing Josephson junctions are steadily improving, but considerable work will have to be done before these techniques mature for industrial scale applications. One intriguing possibility is that on-going breakthroughs in room temperature superconducting (currently possible only at extremely high pressures), may one day enable broad use of superconducting logic for both classical and quantum computers, enabling extremely high switching rates and very low power consumption.
It is not yet clear whether superconducting quantum computers will be the winner in the long term technology race, but quantum computing has already considerably matured superconducting logic technology. New applications for superconducting logic will undoubtedly find a broad range of future applications.
Highlights for the Week
https://semiengineering.com/startup-funding-march-2021/: An interesting roundup of recently funded semiconductor and driverless car startups.
https://www.washingtonpost.com/national-security/china-hypersonic-missiles-american-technology/2021/04/07/37a6b9be-96fd-11eb-b28d-bfa7bb5cb2a5_story.html: Chinese hypersonic missile development programs are leveraging American electronic design automation tools like Synopysys and Cadence.
Subscription, Feedback and Comments
If you liked this post, please consider subscribing! We have weekly subscriber-only posts on Tuesdays.
Please feel free to email me directly (bharath@deepforestsci.com) with your feedback and comments!
About
Deep Into the Forest is a newsletter by Deep Forest Sciences, Inc. We’re a deep tech R&D company specializing in the use of AI for deep tech development. We do technical consulting and joint development partnerships with deep tech firms. Get in touch with us at partnerships@deepforestsci.com! We’re always welcome to new ideas!
Credits
Author: Bharath Ramsundar, Ph.D.
Editor: Sandya Subramanian